A One Health perspective on pet parasiticides
13 February 2025
A ‘One Health’ approach to the control of pet parasites considers the broader implications of parasiticide use, balancing animal, human and environmental health. With funding support from a BSAVA PetSavers Clinical Research Project grant, Rosemary Perkins is investigating imidacloprid emissions from collar-treated dogs to assess the significance of this pollution pathway. In this article Dr Perkins explains more about the study and how this research fits into the broader picture of evidence-based and responsible pet parasiticide use.
The concept of One Health recognizes that human well-being, animal health, and environmental integrity are closely linked and interdependent. This holistic perspective is particularly relevant when it comes to the control of parasites affecting pets. Parasiticides used to treat fleas, ticks and worms are important for safeguarding cats and dogs from harmful parasites; however, their impact extends beyond pet health, influencing human safety and environmental sustainability (Figure 1). A One Health perspective considers the wider impact of parasiticide use, balancing efficacy, safety, and ecological responsibility. As much of the existing information and advice focuses on the risks posed by parasites, this article focuses primarily on the risks associated with parasiticide use, identifying key knowledge gaps, describing new research tackling these knowledge gaps and suggesting ways forward to address this complex One Health area.
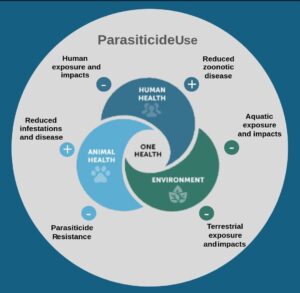
FIGURE 1: One Health schema illustrating the benefits (+) and risks (-) of parasiticide use.
Environmental health
The environmental risk posed by a substance is determined by two main factors: hazard (the potential to cause harm, such as toxicity or persistence in the environment) and exposure (the degree to which organisms or ecosystems encounter the substance).1 Parasiticides are typically both persistent and toxic, making them inherently hazardous and capable of causing environmental harm if they enter ecosystems. Historically, environmental risk assessments for pet parasiticides assumed minimal environmental exposure and thus negligible environmental risk.2 However, evidence of widespread waterway pollution from fipronil and imidacloprid used in pet spot-on treatments3 has challenged this assumption, highlighting the need for a review of the international regulatory framework governing these products.4,5
The historical underestimation of environmental exposure has resulted in limited research into the safety of pet parasiticides, including their environmental pathways, ecotoxicity, and environmental prevalence.5 Parasiticides can enter the environment through multiple routes, depending on factors such as administration method, excretion pathways, product disposal, and human and animal behaviours, such as swimming, handwashing, and disposal of pet faeces. For spot-on treatments, down-the-drain emissions through wastewater from activities like owner handwashing, dog bathing, and the washing of pet bedding are major sources of imidacloprid and fipronil contamination in surface waters, with emissions occurring for at least 28 days after application.3 Evidence of high levels of pollution in dog swimming ponds indicates that direct entry into waterways is a further source of emissions.6 However, parasiticide emissions from other administration methods, such as collars and tablets, remain poorly understood.
Among hundreds of organic chemicals monitored in UK surface waters, fipronil and imidacloprid are among those representing the greatest threat to freshwater ecosystems.7,8 Whilst little research exists on their impact in UK surface waters, research from elsewhere in the world suggests this is likely to be significant. Fipronil and imidacloprid are highly toxic to a wide range of aquatic invertebrates, exerting both lethal and sublethal effects. Notably, several degradates of fipronil are more toxic than the parent compound. Aquatic invertebrates play a vital role in ecosystem functions such as decomposition, nutrient cycling, and serving as a food source for various species.9 Research from the US and Europe has linked these pesticides to the degradation of aquatic biodiversity across multiple organizational levels, from genes to individual organisms and, ultimately, entire ecosystems. Miller et al.10 for instance, found that even low concentrations of fipronil and its degradates negatively impacted stream communities, disrupting emergence patterns in aquatic invertebrates and trophic cascades in the wider food web. Additionally, large-scale studies have shown a significant negative correlation between imidacloprid concentrations and the abundance of macroinvertebrates in freshwater ecosystems,11 with imidacloprid in surface waters also linked to declines in insectivorous bird populations, likely due to cascading trophic effects.12
Due, at least in part, to growing concerns around the safety of fipronil and imidacloprid, there has been a shift in recent years towards a new class of systemic ectoparasiticides known as isoxazolines. Like many orally administered parasiticides (including most anthelmintics), isoxazolines are primarily excreted in the faeces, so it may be possible to limit environmental exposure through appropriate disposal of faeces. However, as with all pet parasiticides, knowledge regarding their environmental fate and ecotoxicity is limited and there are reasons for concern. Soil contamination is still likely to occur for a proportion of treated animals, including outdoor cats. Furthermore, research suggests that isoxazolines may be more potent and persistent than existing parasiticides, including imidacloprid,13 and there is evidence of accumulation in hair and skin,2 suggesting that additional pathways to the household and environment may exist. This raises the possibility of ‘regrettable substitution’ where a cycle of environmental harm is perpetuated through replacement of one chemical with another with unintended similar or worse effects. Research on the comparative environmental toxicity, toxic load, degradation products, excretion routes and environmental fate of pet parasiticides is needed to inform evidence-based treatment decisions that minimize environmental harm whilst delivering treatment efficacy.
BSAVA PetSavers-funded research
We were awarded funding from BSAVA PetSavers for a study to measure imidacloprid emissions from 10 dogs treated with parasiticide collars containing imidacloprid (Figure 2). Bathing, bed washing, and owner handwashing emissions will be measured at regular intervals over 8 months. The contribution of down-the-drain imidacloprid emissions from collar-treated dogs to measured pollution will be calculated to assess the overall significance of this source. Imidacloprid emissions from individual collar-treated dogs will also be compared to those from dogs treated with spot-on formulations, to assess the comparative environmental risks posed by these administration routes and help to inform treatment decisions which consider the broader environmental impact of parasiticide use. Measurement of handwashing emission will also help to assess the extent of human exposure to parasiticides from collar-treated pets.
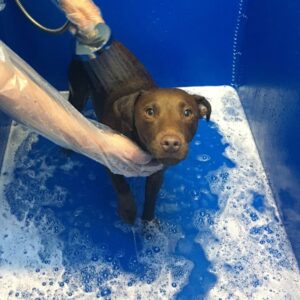
FIGURE 2: BSAVA PetSavers is funding research on parasiticide emissions from collar-treated dogs.
Human health
Given the extensive use of pet parasiticides and contamination of the household with topically applied products, the potential for human exposure to pet parasiticides is considerable. Persistent low-grade exposure to agricultural pesticides has been linked to numerous health concerns, including cardiovascular disease, neurodegenerative disease such as Parkinson’s and neoplasia,14,15 and children are particularly susceptible to the effects of pesticide exposure due to their immature immune system and high metabolism rates.16
Limited information exists regarding the effects of chronic human exposure to pet parasiticides; however, studies have shown an association between fipronil exposure and diabetes and hypertension,17 and the US Environmental Protection Agency has labelled fipronil a potential human carcinogen.18 Due to concerns around human health, fipronil is not authorized for use in food-producing animals, and in 2017 potential health risks associated with fipronil received media prominence following the discovery of fipronil-contaminated eggs in several countries, including the UK, Germany and Switzerland.19 The user risk assessment for fipronil in veterinary medicine concludes that the risk to users is acceptable as the active substance has not shown developmental toxicity.20 However, this assessment does not consider fipronil’s toxic degradation products, and subsequent research suggests developmental toxicity for fipronil sulfone, with adverse health outcomes in newborns associated with in utero exposure to fipronil sulfone including reduced birth weight and thyroid hormone levels.21,22 Fipronil is reported to have a low dermal absorption, with the main toxicity route being through oral exposure; however, little research exists on the pharmacokinetics or health impacts of prolonged dermal exposure.
Due to their relatively recent introduction to the market and limited use beyond the veterinary sector, little is known about the risks posed by isoxazolines to human health. Topical fluralaner, commonly prescribed to cats in veterinary healthcare plans, has a systemic bioavailability of ~25%,23 indicating that a substantial proportion of the administered dose may remain on the coat, where transfer to human hands and subsequent dermal absorption is likely to occur. Furthermore, like many pesticides including fipronil, isoxazolines are classified as per- and polyfluoroalkyl substances (PFAS), due to the presence of fluorinated methyl groups in their chemical structure.24 In recent years, PFAS have received increasing attention due to their widespread environmental presence and potential adverse human health effects. PFAS are a class of synthetic chemicals widely used in various applications owing to their resistance to heat, water, and grease, and are commonly known as ‘forever chemicals’ because of their persistence in the environment.25 The environmental impact of PFAS is a critical concern due to their capacity to accumulate in water, soil and biota, leading to the contamination of drinking water supplies and ecosystems. Their persistence and ubiquity in the environment makes it difficult to undo any pollution, making their management a key priority in environmental science and public.26
The risk to human health from zoonoses such as bartonellosis and toxocariasis is sometimes cited as a reason for blanket (all animals, all year round) parasiticide use. However, zoonotic disease associated with pet parasites is uncommon to rare in the UK,27,28 and unlikely to justify the risks associated with indiscriminate parasiticide use.
Animal health
The benefits of modern parasiticides to animal health — such as ease of use and efficacy — are clear; however, these advantages must be weighed against the risks associated with their use, to ensure their judicious and responsible application. It is beyond the scope of this article to discuss the various parasites affecting cats and dogs; however, it should be noted that understanding the true threat from pet parasites and parasite-related disease remains challenging due to limited and sometimes inconsistent data. For example, Abdullah et al.29 reported that 28.1% of cats and 14.4% of dogs presenting at veterinary practices were infested with fleas. This study (‘The Big Flea Study’) used a citizen science approach, with the selection of cats and dogs performed by participating veterinarians. As the study was primarily designed to survey pathogens in fleas collected from pets and not the prevalence of fleas, it is possible that flea-infested animals were more likely to be selected for inclusion. Indeed, a previous study on ticks utilizing the same methodology concluded that the estimations of prevalence were likely to have been inflated by the method of participant recruitment.30 More recent analysis of veterinary practice electronic health data reported far lower flea infestation rates, with Farrell et al.31 reporting a prevalence of 1.2% in cats and 0.25% in dogs whilst O’Neill et al.32,33 reported a prevalence of 5.1% in cats and 2% in dogs, respectively. It should be noted that some cases of flea infestations may not have been detected or recorded in clinical notes in the latter studies, potentially resulting in an underestimation of flea prevalence. Bond et al.34 reported that almost half of dog and cat owners were unaware of their pet’s flea infestation – demonstrating that some cases may be undetected.
Despite these discrepancies, the importance of effective parasiticides remains undeniable. However, in addition to human health and environmental concerns, their misuse and overuse may result in the development of resistance. For example, exposing fleas to sublethal doses of parasiticides following environmental contamination can select for resistant strains over time.35 The development of resistance within flea populations may be slow because of the presence of large refugia populations, and parasitology researchers have been reluctant to conclude that the reported lack of flea treatment efficacy denotes resistance, generally assuming that treatment failure largely results from operational aspects and treatment deficiencies. However, widespread Ctenophalides felis resistance to older parasiticide compounds such as cyclodienes, carbamates, organophosphates and pyrethroids36 supports the assertion that the onset of resistance to newer products may be inevitable, especially if widely used on a prophylactic basis.37
Indeed, a growing body of research supports the conclusion that flea resistance to parasiticides, especially fipronil, is already present and becoming increasingly common.38-40 Cooper et al.41 found that 62% of cats and 45% of dogs treated with a fipronil-based product still had fleas, reasoning that widespread over-the-counter availability of generic fipronil-based products may have contributed to the selection for resistance. More recently, Ramon-Portugal et al.42 described a mechanism of metabolic resistance to fipronil through increased detoxification in a resistant flea strain.
Surveillance of parasiticide resistance and the development of management strategies to minimize its emergence are vital to protect the efficacy of parasiticides, yet little independent monitoring exists. Mitigation strategies may include methods such as rotating parasiticides and promoting strategies that reduce reliance on synthetic pesticides altogether. Integrated pest management is an approach to pest control that aims to minimize the impact of pests while reducing reliance on pesticides through monitoring, targeted treatment, and sustainable non-chemical methods.43 In the context of pet ectoparasites, this could involve methods such as regular flea combing for early detection of infestations, regular vacuuming and bed washing and targeted treatment based on diagnosis or risk assessment.
Whilst studies indicate reduced efficacy for some parasiticides in recent years, especially fipronil, suggesting the development of resistance, rapid efficacy has been demonstrated for the newer generation of isoxazoline parasiticides. Williams et al.44 found that oral treatment of dogs was highly effective for eliminating fleas in a simulated flea-infested home environment, controlling egg laying, larval development and flea reproduction even at sub-insecticidal concentrations, and providing over 99% flea-control efficacy on dogs from day 1 through 12 weeks post-administration. Likewise, lotilaner achieved efficacy rates of 99.1%, 99.5%, 99.9%, and 99.8% on days 14, 28, 56 and 84, respectively, in client-owned dogs.45 These findings indicate that isoxazolines should provide swift and effective resolution of flea infestations, provided resistance is avoided – weakening the ‘prevention is better than cure’ argument for flea control. Additionally, mechanical methods, such as the My Flea Trap™, have been shown to effectively reduce environmental infestations, trapping over 90% of fleas released into a room.46
Conclusion and ways forward
The BVA, BSAVA and BVZSA have published a joint policy position on responsible parasiticide usage in cats and dogs,47 which highlights some of the concerns and knowledge gaps surrounding this subject and advises a risk-based approach to parasiticides that avoids blanket treatment of pets. Instead, risk assessments on individual animals considering various factors such as lifestyle and season are encouraged, and veterinary businesses are advised not to have blanket treatment policies in place, instead empowering individual vets to have those conversations with their client. However, the statement acknowledges that significant knowledge gaps regarding the risks associated with both parasites and parasiticides make this assessment difficult. Further research on the environmental fate and ecotoxicity of pet parasiticides, as well as the prevalence and severity of parasite-associated disease is needed. By supporting research on parasiticide emissions from collar-treated dogs, BSAVA PetSavers is helping to address these knowledge gaps.
Tools using electronic health data to provide an indication of risk, such as the University of Liverpool’s ‘Flea activity index’ which maps flea activity across the UK,48 can also support evidence-based decision-making to enable a more integrated approach to the control of parasites. Following the publication of the BVA’s position statement, a number of risk assessment tools have been developed by parasiticide manufacturers. Whilst these may help to provide an indication of parasite risk, a lack of transparency regarding the data on which the assessments are based and the exclusion of key risk factors such as seasonality and relative species risk limits their usefulness.
Much of the prevailing narrative in this area has focused on the risks associated with parasites. Only recently has attention also been given to drawbacks of pet parasiticide use. Arguably, routine treatment for pet parasites, initially based on a presumption of negligible risk, is now embedded in many veterinary business models in the form of healthcare plans which include year-round prophylactic parasiticide coverage, and in the expectations of pet owners. However, growing unease surrounding large scale pet parasiticide usage has led to the emergence of green healthcare plans, which encourage parasite monitoring instead of treatment, demonstrating that such plans can be offered without the routine inclusion of parasiticides.49
A significant challenge currently confronting the veterinary profession is the need to provide balanced, interdisciplinary information and guidance on the responsible use of pet parasiticides, free from conflicts of interest. This guidance must cater not only to veterinary professionals but also to pet owners. Presently, much of the available guidance from parasiticide industry-funded sources continues to advocate for year-round blanket treatment of parasites such as fleas, often with little provision of information on the risks associated with parasiticide use.50,51
Given the increasing emphasis on contextualized care in veterinary practice, it is important to provide pet owners with balanced, evidence-based information about parasiticides and choices regarding their use. Educating owners on both the benefits and risks of parasiticides is essential, empowering them to make informed decisions. This education should also include the option to monitor for parasites as an alternative to routine preventative treatment, ensuring that owners are equipped with the necessary information to make informed choices.
To effectively address the multifaceted issue of parasite control in pets, an interdisciplinary One Health approach which integrates insights and expertise from various disciplines, including veterinary medicine, environmental science and public health, is needed to develop sustainable, long-term solutions. By doing so, we can better balance and optimize the health of pets, people and the environment.
About the author
Supporting more BSAVA PetSavers research
BSAVA PetSavers is supported solely by charitable donations. Help us fund more clinically-relevant veterinary research by donating today at www.bsava.com/petsavers/donate.
References
- Lofstedt RE (2011) Risk versus hazard – how to regulate in the 21st century. European Journal of Risk Regulation 2(2), 149–168; doi: 10.1017/S1867299X00001033.
- European Medicines Agency (2000) CVMP/VICH/592/98 – FINAL. VICH Topic GL 6 (Exociticity phase 1), p. 4. Available at: https://www.ema.europa.eu/en/documents/scientific-guideline/vich-gl6-environmental-impact-assessment-eias-veterinary-medicinal-products-phase-i-step-7_en.pdf.
- Perkins R, Barron L, Glauser G, Whitehead M, Woodward G and Goulson D (2024) Down-The-Drain Pathways for Fipronil and Imidacloprid Applied as Spot-on Parasiticides to Dogs: Estimating Aquatic Pollution. Science of the Total Environment 917; doi: https://doi.org/10.1016/j.scitotenv.2024.170175
- VMD (Veterinary Medicines Directorate) (2024) Pharmaceuticals in the Environment (PiE). Available at: https://www.vmdconnect.uk/the-environment (Accessed: 12 August 2024)
- EMA (European Medicines Agency) (2023) Reflection paper on the environmental risk assessment of ectoparasiticidal veterinary medicinal products used in cats and dogs, 31(December), 1–63. Available at: https://www.ema.europa.eu/en/documents/scientific-guideline/reflection-paper-environmental-risk-assessment-ectoparasiticidal-veterinary-medicinal-products-used-cats-and-dogs_en.pdf
- Yoder LE, Egli M, Richardson AK et al. (2024) Dog swimming and ectoparasiticide water contamination in urban conservation areas: A case study on Hampstead Heath, London. Science of the Total Environment. Elsevier BV, 955(October), p. 176686; doi: 10.1016/j.scitotenv.2024.176686
- Spurgeon D, Wilkinson H, Civil W et al. (2022) Worst-case ranking of organic chemicals detected in groundwaters and surface waters in England. Science of the Total Environment. Elsevier BV, 835(March), p. 155101; doi: 10.1016/j.scitotenv.2022.155101
- Egli M, Rapp-Wright H, Oloyede O et al. (2023) A One-Health environmental risk assessment of contaminants of emerging concern in London’s waterways throughout the SARS-CoV-2 pandemic. Environment International 180(August); doi: 10.1016/j.envint.2023.108210
- Macadam CR and Stockan JA (2015) More than just fish food: Ecosystem services provided by freshwater insects. Ecological Entomology 40(S1), 113–123; doi: 10.1111/een.12245
- Miller JL, Schmidt TS, Van Metre PC et al. (2020) Common insecticide disrupts aquatic communities: A mesocosm-to-field ecological risk assessment of fipronil and its degradates in U.S. streams. Science Advances. American Association for the Advancement of Science 6(43), p. eabc1299; doi: 10.1126/sciadv.abc1299
- Van Dijk TC, Van Staalduinen MA and Van der Sluijs JP (2013) Macro-Invertebrate Decline in Surface Water Polluted with Imidacloprid, PLoS ONE. Public Library of Science 8(5); doi: 10.1371/journal.pone.0062374
- Hallmann CA, Foppen RPB, van Turnhout CAM, de Kroon H and Jongejans E (2014) Declines in insectivorous birds are associated with high neonicotinoid concentrations. Nature 511(7509), 341–343; doi: 10.1038/nature13531
- Burgess ER, Geden CJ, Lohmeyer KH, King BH, Machtinger ET and Scott JG (2020) Toxicity of fluralaner, a companion animal insecticide, relative to industry-leading agricultural insecticides against resistant and susceptible strains of filth flies. Scientific Reports. Nature Publishing Group UK 10(1), 1–8; doi: 10.1038/s41598-020-68121-z
- Kim KH, Kabir E and Jahan SA (2017) Exposure to pesticides and the associated human health effects. Science of the Total Environment. Elsevier BV 575, 525–535; doi: 10.1016/j.scitotenv.2016.09.009
- Mostafalou S and Abdollahi M (2017) Pesticides: an update of human exposure and toxicity. Archives of Toxicology. Springer Berlin Heidelberg 91(2), 549–599; doi: 10.1007/s00204-016-1849-x
- Iglesias-González A, Hardy EM and Appenzeller BMR (2020) Cumulative exposure to organic pollutants of French children assessed by hair analysis, Environment International 134, 105332; doi: 10.1016/j.envint.2019.105332
- Peng FJ, Lin CA, Wada R et al. (2024) Association of hair polychlorinated biphenyls and multiclass pesticides with obesity, diabetes, hypertension and dyslipidemia in NESCAV study. Journal of Hazardous Materials 461, 132637; doi: 10.1016/j.jhazmat.2023.132637
- Hurley P (1998) Mode of carcinogenic action of pesticides inducing thyroid follicular cell tumors in rodents. Environ Health Perspect 106(8); doi: 10.1289/ehp.98106437
- BBC (2017) Eggs containing fipronil found in 15 EU countries and Hong Kong. Available at: https://www.bbc.co.uk/news/world-europe-40896899
- EMA European Medicines Agency (2013) CVMP assessment report Broadline. Available at: https://www.ema.europa.eu/en/documents/assessment-report/broadline-epar-public-assessment-report_en.pdf
- Kim YA, Yoon YS, Kim HS et al.(2019) Distribution of fipronil in humans, and adverse health outcomes of in utero fipronil sulfone exposure in newborns, International Journal of Hygiene and Environmental Health 222(3), 524–532; doi: 10.1016/j.ijheh.2019.01.009
- Béranger R, Hardy EM, Binter AC et al. (2020) Multiple pesticides in mothers’ hair samples and children’s measurements at birth: Results from the French national birth cohort (ELFE). International Journal of Hygiene and Environmental Health 223(1), 22–33; doi: 10.1016/j.ijheh.2019.10.010
- Kilp S, Famirez D, Allan MJ and Roepke RKA (2016) Comparative pharmacokinetics of fluralaner in dogs and cats following single topical or intravenous administration. Parasites and Vectors 9(1), 1–7; doi: 10.1186/s13071-016-1564-8
- Wang Z, Buser AM, Cousins IT et al. (2021) A New OECD Definition for Per- And Polyfluoroalkyl Substances. Environmental Science and Technology 55(23), 15575–15578; doi: 10.1021/acs.est.1c06896
- Brunn H, Arnold G, Körner W, Rippen G, Steinhäuser KG and Valentin I (2023) PFAS: forever chemicals—persistent, bioaccumulative and mobile. Reviewing the status and the need for their phase out and remediation of contaminated sites. Environmental Sciences Europe 35(1), 1–50; doi: 10.1186/s12302-023-00721-8
- Wang Z, DeWitt JC, Higgins CP and Cousins IT (2017) A Never-Ending Story of Per- and Polyfluoroalkyl Substances (PFASs)? Environmental Science and Technology 51(5), 2508–2518; doi: 10.1021/acs.est.6b04806
- Chaloner GL, Harrison TG, Coyne KP, Aanensen DM and Birtles RJ (2011) Multilocus sequence typing of Bartonella henselae in the United Kingdom indicates that only a few, uncommon sequence types are associated with zoonotic disease. Journal of Clinical Microbiology 49(6), 2132–2137; doi: 10.1128/JCM.00275-11
- Patterson J (2022) Toxocarosis in humans: How much of a problem is it in the UK? Drug and Therapeutics Bulletin 61(1), 7–11; doi: 10.1136/dtb.2022.000052
- Abdullah S, Helps C, Tasker S, Newbury H and Wall R (2019) Pathogens in fleas collected from cats and dogs: distribution and prevalence in the UK. Parasites & Vectors 12(71); doi: 10.1186/s13071-019-3326-x
- Abdullah S, Helps C, Tasker S, Newbury H and Wall R (2016) Ticks infesting domestic dogs in the UK: A large-scale surveillance programme. Parasites & Vectors 9(1), 391; doi: 10.1186/s13071-016-1673-4
- Farrell S, McGarry J, Noble PJM et al. (2023) Seasonality and other risk factors for fleas infestations in domestic dogs and cats. Medical and Veterinary Entomology 37(2), 359–370; doi: 1111/mve.12636
- O’Neill DG, Gunn-Moore D, Sorrell S et al. (2023) Commonly diagnosed disorders in domestic cats in the UK and their associations with sex and age. Journal of Feline Medicine and Surgery 25(2); doi: 10.1177/1098612X231155016
- O’Neill DG, James H, Brodbelt DC, Church DB and Pegram C (2021) Prevalence of commonly diagnosed disorders in UK dogs under primary veterinary care: results and applications. BMC Veterinary Research 17(1), 1–14; doi: 10.1186/s12917-021-02775-3
- Bond R, Riddle A, Beugnet F and Stevenson R (2007) Survey of flea infestation in dogs and cats in the United Kingdom during 2005. Veterinary Record 160(15), 503–506; doi: 10.1136/vr.160.15.503
- Bossard R, Hinkle NC and Rust MK (1998) Review of insecticide resistance in cat fleas (Siphonaptera: Puhcidae). Journal of Medical Entomology 35(4), 415–422; doi: 10.1093/jmedent/35.4.415
- Halos L, Beugnet F, Cardoso L et al. (2014) Flea control failure? Myths and realities, Trends in Parasitology 30(5), 228–233; doi: 10.1016/j.pt.2014.02.007
- Coles TB and Dryden MW (2014) Insecticide/acaricide resistance in fleas and ticks infesting dogs and cats. Parasites & Vectors 7(1), 1–10; doi: 10.1186/1756-3305-7-8
- Fisara P, Guerino F and Sun F (2019) Efficacy of a spot-on combination of fluralaner plus moxidectin (Bravecto® Plus) in cats following repeated experimental challenge with a field isolate of Ctenocephalides felis. Parasites & Vectors 12(1), 1–7; doi: 10.1186/S13071-019-3512-X/FIGURES/1
- Dryden MW, Payne PA, Smith V et al. (2013) Evaluation of indoxacarb and fipronil (s)-methoprene topical spot-on formulations to control flea populations in naturally infested dogs and cats in private residences in Tampa FL. USA. Parasites & Vectors. 6(1), 1–7; doi: 10.1186/1756-3305-6-366/TABLES/2
- Wong S, Elsheikha H and Dryden M (2021) Flea product efficacy, pet owners’ adherence and treatment failure: what’s the connection? Companion Animal 26(8), 182–190; doi: 10.12968/COAN.2021.0006
- Cooper AR, Nixon E, Vineer HR, Abdullah S, Newbury H and Wall R (2020) Fleas infesting cats and dogs in Great Britain: spatial distribution of infestation risk and its relation to treatment. Medical and Veterinary Entomology 34(4), 452–458; doi: 10.1111/mve.12462
- Ramon-Portugal F, Liénard E, Legeay A, Lacroix M and Bouhsira E (2023) Flea resistance to fipronil: Confirmation of metabolic detoxification through innovative HPLC dosage within flea. Journal of Veterinary Pharmacology and Therapeutics; doi: https://onlinelibrary.wiley.com/doi/10.1111/jvp.13193
- European Commission (2023) Integrated Pest Management. Available at: https://food.ec.europa.eu/plants/pesticides/sustainable-use-pesticides/integrated-pest-management-ipm_en (Accessed: 20 March 2024)
- Williams H, Yound DR, Qureshi T, Zoller H and Heckeroth AR (2014) Fluralaner, a novel isoxazoline, prevents flea (Ctenocephalides felis) reproduction in vitro and in a simulated home environment. Parasites & Vectors 7(1), 275; doi: 10.1186/1756-3305-7-275
- Cavalleri D, Murphy M, Seewald W, Drake J and Nanchen S (2017) A randomised, blinded, controlled field study to assess the efficacy and safety of lotilaner tablets (CredelioTM) in controlling fleas in client-owned dogs in European countries. Parasites & Vectors 10(1), 1–8; doi: 10.1186/s13071-017-2479-8
- Müller GC, Dryden MW, Revay EE et al. (2011) Understanding attraction stimuli of the cat flea, Ctenocephalides felis, in non-chemical control methods. Medical and Veterinary Entomology 25(4), 413–420; doi: 10.1111/j.1365-2915.2011.00960.x
- BVA (British Veterinary Association) (2021) BVA , BSAVA and BVZS policy position on responsible use of parasiticides for cats and dogs. Available at: https://www.bva.co.uk/media/4352/bva-bsava-and-bvzs-policy-position-on-responsible-use-of-parasiticides-for-cats-and-dogs.pdf
- SAVSNET (no date) Flea activity dashboard (2023) Available at: https://public.tableau.com/app/profile/savsnet.at.liverpool/viz/Fleaactivitydashboard/fleadashboard
- VetSustain (2024) Small World Vet Centre: A Sustainable Approach to Clinical Veterinary Practice. Available at: https://vetsustain.org/work/small-world-vet-centre
- Elanco (2024) Top flea prevention tips every pet owner must know. Available at: https://mypetandi.elanco.com/en_gb/parasites/fleas/top-flea-prevention-tips (Accessed: 3 March 2024)
- Wright I (2024) Parasite prevention: what, where, why and when? Vet Times. Available at: https://www.vettimes.co.uk/article/parasite-prevention-what-where-why-and-when/